So here’s something intriguing: an observational signature from the very first stars in the universe, which formed about 180 million years after the Big Bang (a little over one percent of the current age of the universe). This is exciting all by itself, and well worthy of our attention; getting data about the earliest generation of stars is notoriously difficult, and any morsel of information we can scrounge up is very helpful in putting together a picture of how the universe evolved from a relatively smooth plasma to the lumpy riot of stars and galaxies we see today. (Pop-level writeups at The Guardian and Science News, plus a helpful Twitter thread from Emma Chapman.)
But the intrigue gets kicked up a notch by an additional feature of the new results: the data imply that the cosmic gas surrounding these early stars is quite a bit cooler than we expected. What’s more, there’s a provocative explanation for why this might be the case: the gas might be cooled by interacting with dark matter. That’s quite a bit more speculative, of course, but sensible enough (and grounded in data) that it’s worth taking the possibility seriously.
[Update: skepticism has already been raised about the result. See this comment by Tim Brandt below.]
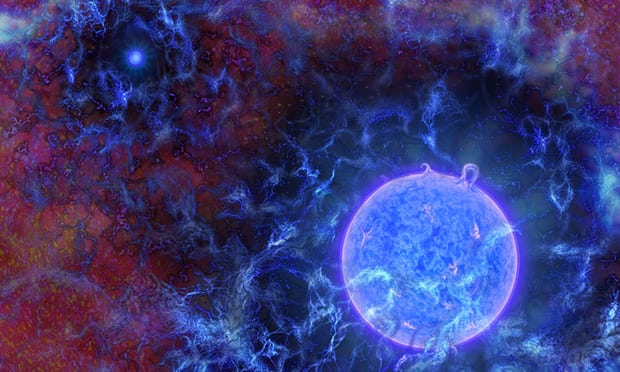
Let’s think about the stars first. We’re not seeing them directly; what we’re actually looking at is the cosmic microwave background (CMB) radiation, from about 380,000 years after the Big Bang. That radiation passes through the cosmic gas spread throughout the universe, occasionally getting absorbed. But when stars first start shining, they can very gently excite the gas around them (the 21cm hyperfine transition, for you experts), which in turn can affect the wavelength of radiation that gets absorbed. This shows up as a tiny distortion in the spectrum of the CMB itself. It’s that distortion which has now been observed, and the exact wavelength at which the distortion appears lets us work out the time at which those earliest stars began to shine.
Two cool things about this. First, it’s a tour de force bit of observational cosmology by Judd Bowman and collaborators. Not that collecting the data is hard by modern standards (observing the CMB is something we’re good at), but that the researchers were able to account for all of the different ways such a distortion could be produced other than by the first stars. (Contamination by such “foregrounds” is a notoriously tricky problem in CMB observations…) Second, the experiment itself is totally charming. EDGES (Experiment to Detect Global EoR [Epoch of Reionization] Signature) is a small-table-sized gizmo surrounded by a metal mesh, plopped down in a desert in Western Australia. Three cheers for small science!
But we all knew that the first stars had to be somewhen, it was just a matter of when. The surprise is that the spectral distortion is larger than expected (at 3.8 sigma), a sign that the cosmic gas surrounding the stars is colder than expected (and can therefore absorb more radiation). Why would that be the case? It’s not easy to come up with explanations — there are plenty of ways to heat up gas, but it’s not easy to cool it down.
One bold hypothesis is put forward by Rennan Barkana in a companion paper. One way to cool down gas is to have it interact with something even colder. So maybe — cold dark matter? Barkana runs the numbers, given what we know about the density of dark matter, and finds that we could get the requisite amount of cooling with a relatively light dark-matter particle — less than five times the mass of the proton, well less than expected in typical models of Weakly Interacting Massive Particles. But not completely crazy. And not really constrained by current detection limits from underground experiments, which are generally sensitive to higher masses.
The tricky part is figuring out how the dark matter could interact with the ordinary matter to cool it down. Barkana doesn’t propose any specific model, but looks at interactions that depend sharply on the relative velocity of the particles, as . You might get that, for example, if there was an extremely light (perhaps massless) boson mediating the interaction between dark and ordinary matter. There are already tight limits on such things, but not enough to completely squelch the idea.
This is all extraordinarily speculative, but worth keeping an eye on. It will be full employment for particle-physics model-builders, who will be tasked with coming up with full theories that predict the right relic abundance of dark matter, have the right velocity-dependent force between dark and ordinary matter, and are compatible with all other known experimental constraints. It’s worth doing, as currently all of our information about dark matter comes from its gravitational interactions, not its interactions directly with ordinary matter. Any tiny hint of that is worth taking very seriously.
But of course it might all go away. More work will be necessary to verify the observations, and to work out the possible theoretical implications. Such is life at the cutting edge of science!
Aside from this newest speculation, that Cold Dark Matter, just out of the fridge, presumably, cooled down the universe, do we have any proof that there was Dark Matter then? (And thus that Dark Matter is not emergent?)
Plenty of evidence there was already dark matter then, from fluctuations in the CMB.
Let us hope this puts the idea of monochromatic mass distributions of black holes (upon which most constraints against black hole dark matter are based) to rest once and for all. Black holes were known to merge since long before LIGO, but the LIGO mergers have done wonders for the acceptance of platykurtic black hole mass distributions. Having more black holes without obvious accretion disks is advantageous to humanity as a species, because it vastly increases our long-range colonization options. On the topic, I like e.g. arxiv 1709.07467, 1605.04023, 1701.02544, 1711.06129, 1703.04825, 1702.08275, 1604.05207, 1001.2308, and the early 2016 observation and subsequent confirmations of the Milky Way’s first intermediate mass black hole CO-0.40-0.22.
Possibly It could involve reporting bias. If the signal had not fluctuated up, we likely would not have heard about it.
3.8 sigma is not that big for such a radical idea. We’ll see.
–Arthur Snyder, SLAC
Wow wow wow. I’ve only read this the once so far, but here are my immediate thoughts:
1 – You write so damned well Sean, so precise and articulate, simple and concise, especially given the complex nature of the content.
2 – Total admiration for you folk at the cutting-edges of science.
3 – Never ceases to amaze me how astonishing it is that we (you – see point 2 above) continue to piece together the puzzle of how it was, and how we got to now.
4 – Equally amazed that from these beginnings we ended up with biology on (at least) this third rock from this sun, and eventually a life-form capable of looking back and figuring all this stuff out (with no god nor magic involved!)
5 – There’s some kind of irony about the ‘faith’ and trust we lay-people adopt in all of this i.e. I simply can’t know if any of this is true. But the process of science, it’s core values, open discussion, peer review etc. secures that faith.
6 – Can’t believe how excited I was to experience, for the very first time, the word ‘somewhen’! Initially I thought: what a clever thing Sean has done, but then google assured me it’s a word. I’ve had my mind-blown by the huge and the small, win/win.
7 – Thanks for writing this blog Sean, and for sharing these wonderful developments.
Chris G (Lewes, UK)
“It will be full employment for particle-physics model-builders.” unfortunately, this is undoubtably true. Sabine Hossenfelder has shown the appropriate contempt for this group and Lee Smolin has correctly deplored the group’s take-over of theory/model-building in academic physics. Theoretical biology is becoming what theoretical physics once was. this is appropriate not only because it is a fascinating subject in its own right (the inanimate physical universe is far simpler than the animate biological universe – as Feynman said “”Imagine how much harder physics would be if electrons had feelings.”), but because biology offers a plethora of experimental data with which to evaluate theoretical speculations, in distinct contrast to foundational physics.
Cool. Thank you for the explanation Dr.Carroll. Well done to the experimenters. Great news.
saw a picture of the detector in an article somewhere. Really elegant idea…hope we get more information on dark matter sooner rather than later.
It is unfortunate that the experiment itself would hypothesize about dark matter interaction rather than let the signal speak for itself. At this point, I’m skeptical of the signal, but *very* skeptical of interaction with a particle we have no direct proof of and where the only indirect proof is via gravitational interaction which plays no role whatsoever in this supposed early gas cooling.
In short, our only evidence for the particle theory of dark matter is indirect through a hypothetical gravitational interaction motivated by a discrepancy in galactic rotation dynamics. To use that motivation to hypothesize about some heretofore non-existent non-gravitational interaction entirely disconnected from the original motivation seems like rank speculation of the worst kind.
Maybe there is some as yet undiscovered particle responsible for the non-gravitational interaction cooling this gas, but how can anyone justify speculating that this undiscovered particle is one and the same as another undiscovered particle whose only known motivation is through a gravitational interaction?
So, “speculation” based on some evidence is bad but speculation based on no evidence is not so bad?
It may be that you, and myself or any other non-experts for that matter, don’t know near enough about the relevant physics to warrant any confidence in what we may think about it compared to what experts think about it.
Thanks for your post Sean. The most likely cause of the low temperatures is Doppler cooling via masers produced by interstellar medium during that epoch. Let us not invoke exotic Dark Matter particles each time we are confronted with an astrophysical puzzle. Sooner or later these below expected temperatures will be cited as evidence for Dark Matter particles.
Darrell, not sure how your read my sentence to imply, “speculation based on no evidence is not so bad?” I’m calling into question unwarranted speculation. If you think it is warranted, by all means feel free to explain why.
I would simply say that if space and time are emergent universal properties, then so are the properties of thermodynamics.
Thank you, Sean, for taking the time to inform us of this important observation. And I doubly thank you for making it understandable to a person like myself – who has a basic science and engineering background but is not even close to having the background necessary to work in theoretical physics and cosmology.
I have a thought. Maybe Dark Matter has “always’ been there, in other words, the Universe is infinite in time and our visible Universe is just a quantum fluctuation in the infinite Universe. In that case the real matter could interact with Dark Matter through simple Newtonian contact- like neutrinos do.
I suspect that the signal isn’t real, or at least not at the level reported. Aaron Parsons of Berkeley has a summary of his thoughts here, and you can see the relevant plot from the discovery paper at that link without a paywall. If the team fits their (complicated and wiggly!) background model without fitting a 21cm trough, they get wiggles with an amplitude of around 150 mK (panel b). It looks a lot like a sinusoid with a “wavelength” of about 13 MHz. Quoting from the paper,
The reference is to this paper by the same team. Go to Figure 4 though, and what do the residuals from the same model look like? A sinusoid, “period” of ~15 MHz and a frequency-dependent amplitude, roughly 50 mK at 110 MHz, where the total signal is 600 K. If we assume that this is a systematic that is a constant fraction of the total signal, we can scale 50 mK at 110 MHz to the total foreground of about 2000 K at 70 MHz, obtaining 160 mK. This hypothesis, that the residual is a constant factor (just under 10^-4) of the measured foregrounds, is consistent with the behavior of the sinusoidal residuals in the 2017 paper.
To my eye then, it looks like the high-band antennas do indeed produce a very similar feature in the residuals, and at almost the same fraction of the foreground signal as that seen in the most recent discovery paper. Whether this is uncorrected beam wobble or something else, I think systematics are the most likely explanation.
On the other hand, if you want to get just a little more speculative, the 78 MHz peak in the quasi-sinusoidal residual in Figure 1 panel b of the recent Nature paper is lower than its neighbors on either side–by around 150 mK. This is almost precisely the level expected for this signal in the standard theory. Note that if we assume the real 21-cm signal to be at a slightly lower frequency, say 70-75 MHz (also more consistent with theory), we can even compensate some of the nu^-2.5 behavior we would otherwise expect in a systematic sinusoid like that from the 2017 paper! So there might be a real signal hiding in there after all, and it might be just what was expected from vanilla theory.
Question: is it possible that Dark Matter is the remains of a preceding universe? Or is it a way that a contemporaneous universe partially interacts with ours? or the remains of a collapsed Black Hole?
Is it possible that the discrepancy in the CMB and local values of the Hubble constant, as recently confirmed by the HST team (A. Reiss et al, 2017), could have something to do with the lower than expected temperature of the cosmic gas?
Shaun, if black holes are dark matter, they are probably distributed like this: https://pbs.twimg.com/media/C8_dIOIV0AEqq06.jpg
“Sabine Hossenfelder has shown the appropriate contempt for this group”
With all due respect to Sabine (with whom I agree more often than not, as my comments on her blog demonstrate (though I think she misunderstands many fine-tuning arguments and attacks a straw man), she is one person and her opinion is not gospel. It needs more than contempt from Sabine to discredit a research programme. (Personally, I think that primordial black holes with a range of masses still have a chance, so I am not a big fan of particle dark matter, but, again, it takes more than a snub from Sabine to rule it out.)
Here’s a preposterous question for you. In thinking about dark matter it occurs to me that in the vacuum there are virtual particles popping into and out of existence all of the time. These particles have mass, so should contribute to the gravitational field, but don’t hang around long enough to interact very much. Where does virtual particle mass fit in the mass budget and is there any way this could be dark matter?
John, that’s a great question, and goes to the heart of the “vacuum catastrophe” a/k/a cosmological constant problem: https://en.wikipedia.org/wiki/Cosmological_constant_problem
Someone will correct me if I’m wrong, but we have to assume that the virtual particles have both positive and negative energy cancelling out the effective mass that might lead to gravitation, because if you don’t assume that then they would have 120 orders of magnitude too much mass to agree with observations.
For a while there was a hypothesis that WIMPs could be, at least in part, the degenerate remnants of evaporated primordial black holes. The idea would be that Hawking radiation does not evaporate a black hole into complete nothingness, because there’s a scale below which an event horizon could operate, and that could leave some mass that would only interact with matter via the tiny classical gravity it still had left. Does this hypothesis carry any weight (heh) these days? I haven’t seen it mentioned in a long time.